I was eating breakfast on a Monday morning at Sears Fine Food in downtown San Francisco, casually watching the local five-day weather forecast on a television screen behind the counter. A little symbol along the bottom showed a happy-looking sun for the rest of the day. Wednesday had a friendly-looking cloud and a few raindrops, and Thursday had a dark, threatening cloud with heavier drops. I knew Thursday’s conditions would be much rougher than the symbol conveyed. I had been studying detailed satellite data and weather models, and they indicated that a major atmospheric river (AR) was likely to hit the city. The symbol was completely inadequate for communicating the threat of the approaching storm.
ARs are essentially rivers of water vapor in the sky that are pushed along by strong, low-altitude winds, sometimes at hurricane speeds. The meteorological community formally defined them only in the early 2010s, after improved satellite imaging and science revealed how these storms can form far out over the remote ocean. They can grow to 2,000 miles long, 500 miles wide and two miles deep by the time they strike the western coasts of continents. An average AR brings far greater rainfall than a typical rain or thunderstorm in those parts of the world, transporting enough vapor to equal 25 times the flow rate of the Mississippi River where it pours into the Gulf of Mexico.
These storms can produce disastrous flooding, including the biggest floods that some areas may see in a century. They can occur in families—a series of storms, as if rolling in on a treadmill. Several times a year they pummel the western coasts of the U.S., Canada, Europe, Africa, South America and New Zealand. They can also reach far inland: the raging terrestrial rivers that tore apart roads in Yellowstone National Park and forced the park to close this past June were fueled in large part by a remarkably strong AR.
ARs are not always destructive; sometimes they bring welcome rain to parched regions. They can beneficially boost snowpack and help fill natural and human-made water reservoirs. It’s hard to know more than a few days ahead where exactly a storm will make landfall, however. As big as they are, they can be fickle because numerous forces can affect their progression, from ocean-surface temperatures to pockets of cold air aloft.
On the same day that the AR was slated to hit San Francisco, I was scheduled to present new insights into these storms at the 2016 American Geophysical Union meeting there. Struck by the inadequacy of the TV weather icons, I pledged to finish an intensity scale for ARs—a forecasting and communications tool that colleagues and I had been discussing for a while. Rather than a simplistic icon of a sun or cloud, I envisioned a square, yellow box on the screen for Thursday with a storm system’s ranking in bold, black characters, similar to how hurricanes are characterized as categories 1 to 5. This ranking would allow weather forecasters, emergency planners, safety personnel and reservoir managers—as well as the public—to better prepare for potential flooding, transportation disruptions, downed power lines, debris flows or evacuations.
National Weather Service (NWS) forecasters provide the formal watches and warnings that trigger actions by numerous local officials. That week in San Francisco some of the experts were tuning in to a novel set of AR forecasting tools developed and provided by the Center for Western Weather and Water Extremes (CW3E) at the Scripps Institution of Oceanography, part of the University of California, San Diego, where I work. The tools track 10 or more variables, including the winds a few thousand feet aloft, along with horizontal movement of water vapor, that show the odds of an AR hitting a stretch of the coast. To make a clear and convincing ranking system, we would need to simplify those 10 variables into two quantities that represent the intensity and duration of an incoming AR.
The scale we devised runs from AR1 (primarily beneficial precipitation) to AR5 (primarily hazardous). The goal is to prompt people to pay close attention to the initial intensity level and any subsequent changes because strong ARs can wallop a coastal or inland mountain region with enormous amounts of rain or snow—for hours to days running—forcing ground personnel to regularly reassess actions.
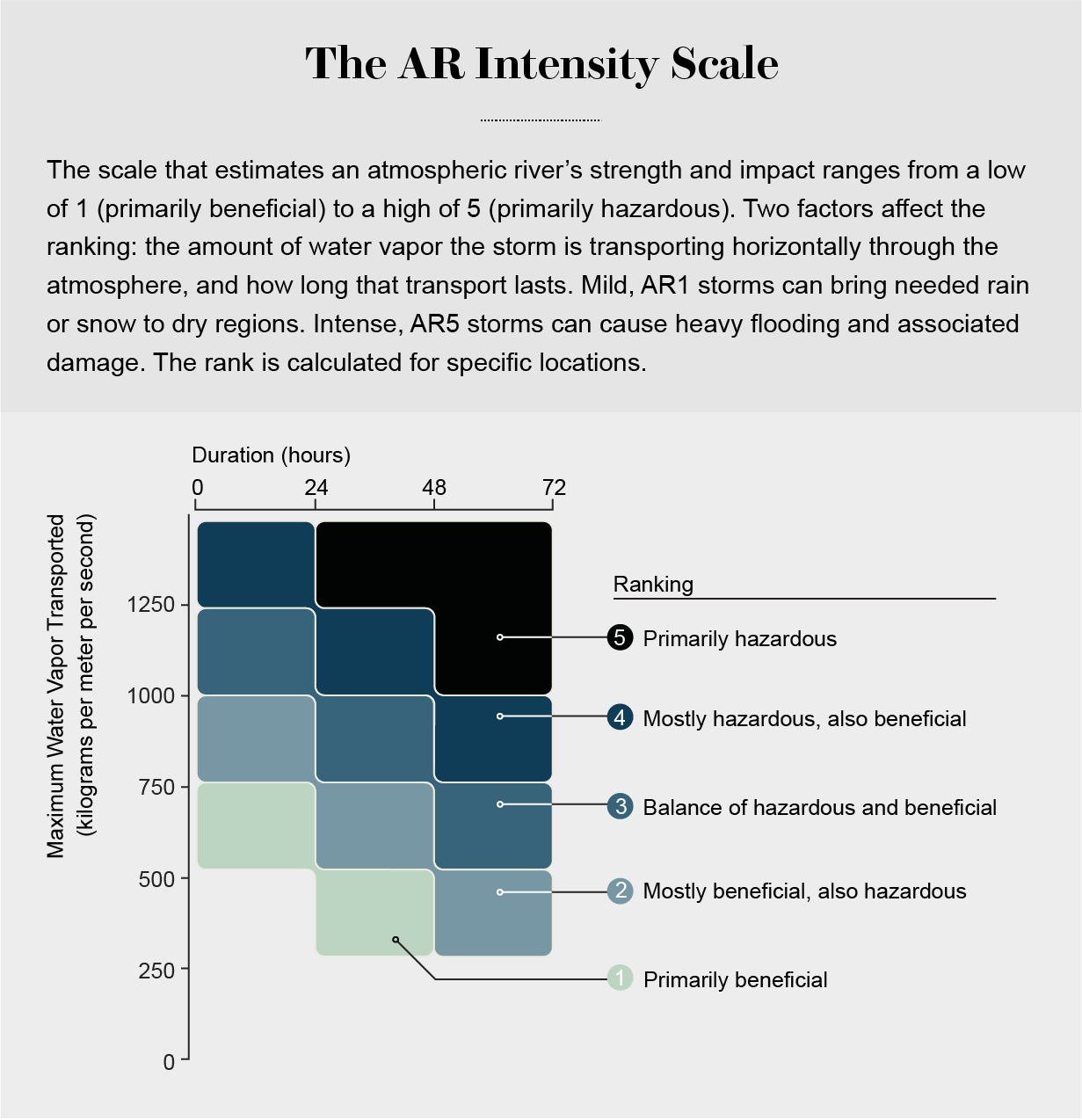
Our team published the AR Scale in the Bulletin of the American Meteorological Society in 2019. A storm’s ranking on the scale reflects a huge amount of work that happens behind the scenes every time scientists first see that an AR is forming. Case studies of several strong ARs that battered the U.S. West Coast over the past two years show how researchers and weather forecasters have gotten better at informing emergency planners and water managers on whether a storm will bring rain that is good for replenishing water supplies or crosses a threshold into floods and landslides.
With greater preparedness, officials can lessen risk to property and lives and know how to maximize water storage. A January 2021 AR that caused heavy landslides that severed cliffside roads along California’s Big Sur coast—yet did not cause a single death—shows how well officials can use the latest forecasting science to observe these storms and manage consequences. The knowledge, forecasts and outcomes in this case illustrate the full potential of better forecasting and communication to help western coasts everywhere be ready for large storms.
Saving Lives
In late January 2021 researchers could see that atmospheric patterns over the Pacific Ocean were setting up to bring another extreme event to the North American West Coast. The question was where an AR would land and how intense it would be. Tension among researchers rose quickly; along certain parts of the coast, as in Mediterranean climates globally, water is often a scarce resource, so residents, farmers and business owners all welcome rain. But of course, flooding rain can ruin agriculture, infrastructure and lives.
In the Pacific Northwest, rivers were still running high after weeks of heavy rains. The last thing people there needed was another wet storm. In California, which had been distressingly dry, a few inches of rain would bring needed relief, but a soaking could cause landslides on steep mountain slopes already stripped bare by wildfire.
On January 20 a team of atmospheric scientists, meteorologists, aircraft crews and staff at the Interagency Atmospheric River Reconnaissance Command Center based at CW3E decided to send data-gathering aircraft into the coalescing storm. Although satellite and ocean-buoy data are crucial to weather models, they do not adequately monitor AR position, strength or water-vapor content, partly because the cloudy or rainy conditions associated with ARs can block satellite data gathering or make it less accurate. Reconnaissance flights fill these gaps.
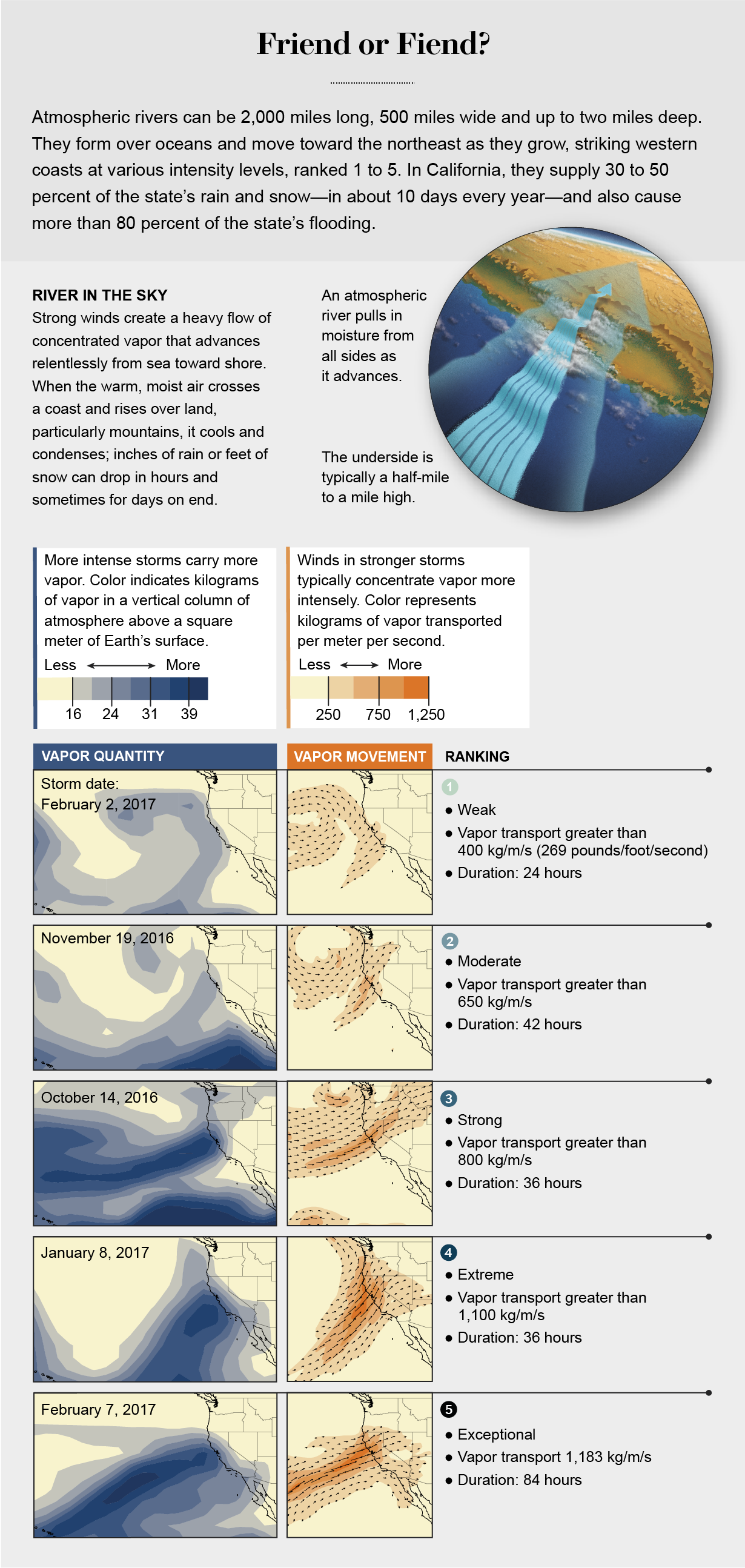
Two days later a G-IV jet flown by the National Oceanic and Atmospheric Administration climbed to 40,000 feet above the ocean west of Hawaii. Every 10 minutes or so, for several hours, it released dropsondes—small instruments that drift down by parachute for 20 minutes or so and measure wind speed and direction, water vapor, temperature and pressure. The instruments radioed data back to the aircraft, which sent it to the global weather data hub, where weather forecasting models draw from to start the next forecast, typically every six or 12 hours. The jet also operated a prototype radio-occultation sensor that uses GPS satellite signals to sense atmospheric conditions as far as 180 miles to the side of the aircraft. Air-pressure data from roughly 100 drifting ocean buoys deployed with the Global Drifter Program were coming into the hub as well.
To the relief of people in the soaked Pacific Northwest, data from the flight helped to predict that the AR would make landfall farther south. But would the rainfall be good for drought-stricken California, or would it be intense and dangerous?
Late on January 22, CW3E analysis indicated that the storm intensity would likely be AR1. We began sending text or e-mail notifications to regional experts who, while also using standard NWS forecast information, would advise civic leaders responsible for actions on the ground. In the mountains above Santa Cruz, where very recent wildfires had scoured steep slopes, fire chiefs began to prepare for landslides. Transportation managers in Big Sur prepared for road closures in case of landslides along Highway 1, where some slopes tower 1,000 feet above the Pacific Ocean.
At the San Francisco Bay Area offices of the U.S. Army Corps of Engineers and at the Sonoma Water Agency, a local utility, reservoir operators used CW3E’s tools and NWS forecasts to determine how much flow from the Russian River and tributaries into Lake Mendocino, a large reservoir, would be beneficial and how much could cause flooding. If the predicted rainfall was modest, they could keep reservoir gates closed to retain the most water possible for the upcoming dry season.
On January 23 we sent the G-IV jet northwest of Hawaii to measure the now mature but slow-moving, low-pressure system, which was pumping water vapor and heat northward into the path of an incoming trough of low pressure moving quickly eastward from Japan and Siberia. The interaction would influence where the AR would form and how it would track in the next few days. CW3E’s tools were indicating the storm could reach AR2 in California, raising the stakes for everyone on the coast.
This higher ranking prompted us to conduct more frequent flights, including two C-130 weather reconnaissance aircraft from the U.S. Air Force Reserve base for AR Recon on the West Coast. By January 24 the forecasts were predicting the AR could stall near Point Conception, north of Los Angeles. But some ensembles—weather models that make multiple runs from the same starting time but with small variations in the starting conditions—showed the storm could stall farther north, near Big Sur, where the fires had been worst. NWS forecasters issued a warning, which in part said: “The AR boundary and associated heavy rainfall will stall over the far southern portion of our forecast area [near Point Conception] Wednesday afternoon before slowly lifting back to the north Wednesday night into early Thursday…. excessive rain rates are possible across any of our burn scars, including … the SCU Fire Complex on Wednesday morning…. In the hills, rain totals are projected to range from 4 to 7 inches, except as much as 9 inches in the Santa Cruz Mountains and as much as 11 inches in the Santa Lucia Mountains.”
By January 26 the AR was aiming between Point Conception and San Jose. AR Recon flew more missions every day, firming up indications that the storm would reach AR2 or AR3 levels and would stall, which meant some unlucky coastal region could experience heavy rain for up to a day and a half. Emergency preparedness officials prepositioned equipment and staff for possible rescues along the burn areas around Monterey and Santa Cruz, as well as the vulnerable Highway 1 along Big Sur.
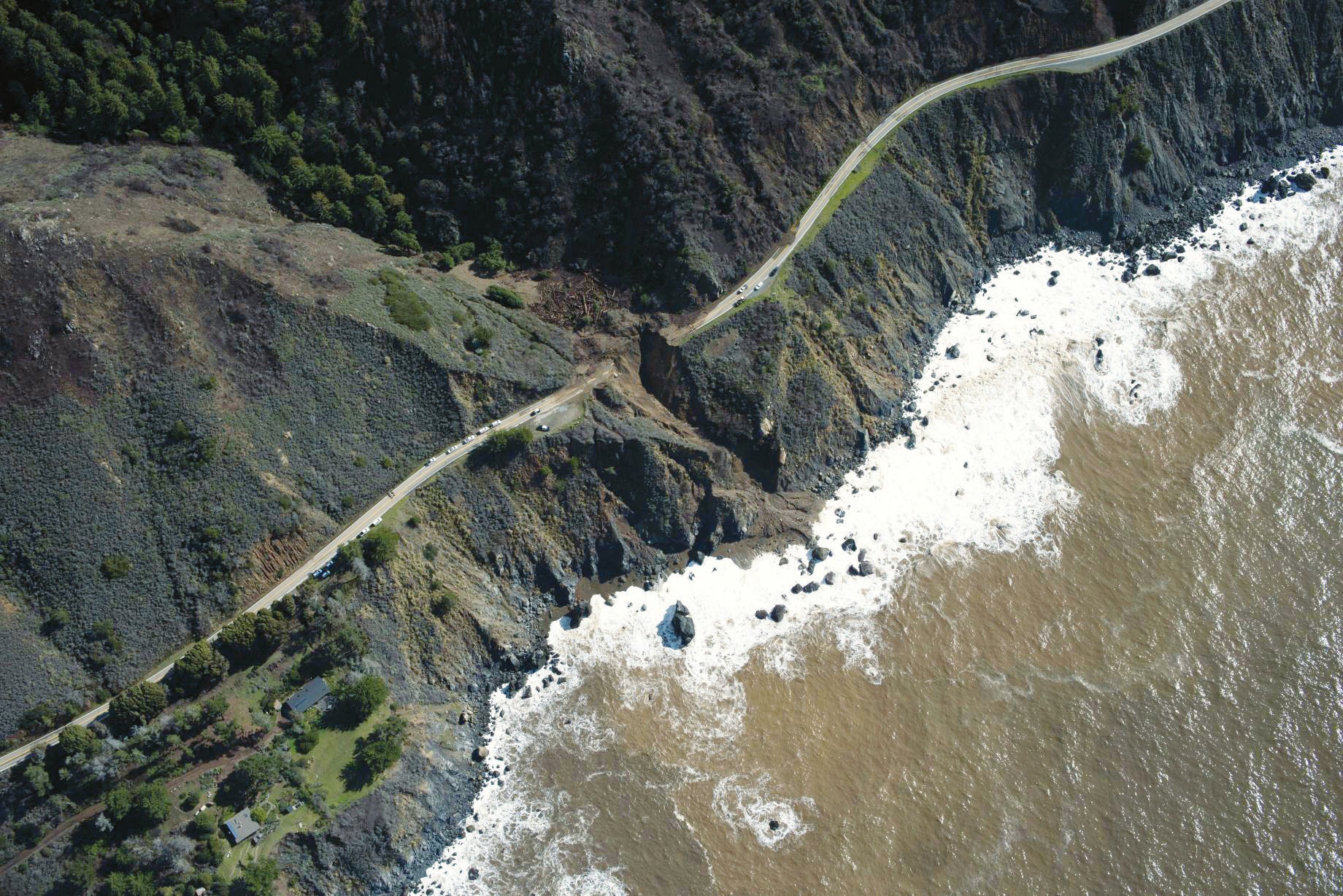
Early on January 27 showers came into view of the NWS “NEXRAD” weather radar network. As predicted, the first in a series of storms struck north of the Bay Area but moved through there quickly. An AR observatory on the ground near Lake Mendocino—which uses radar that looks straight up—confirmed for forecasters and reservoir operators that the main event was going to be south of them. The storms produced just two to three inches of rain over the lake, providing largely beneficial inflow.
Sure enough, the storm stalled as an AR3 near Big Sur and dropped more than 10 inches of rain over two days. Even more fell in the nearby Santa Lucia Mountains. The downpours caused urban flooding as well as heavy debris flows on fire-burned hillsides, destroying homes and commercial structures and severely damaging the vital Highway 1 along the coast. The road ended up being closed for months for significant repairs, disrupting transportation and the local economy. The event became one of the nation’s “billion-dollar weather disasters” of 2021.
Critically, no lives were lost. Preliminary analysis has shown that AR Recon data improved the prediction and reduced the precipitation forecast error by as much as 50 percent; without it, the predicted rain amount for Big Sur would have been much less than what fell. This contributed to accurate warnings and emergency responses.
Warn the World
With climate change bringing more extreme rain events, the U.S. federal government is taking greater notice of ARs. In December 2021 NOAA’s Science Advisory Board sent a formal set of recommendations for the next decade, “Priorities for Weather Research,” to policy makers in Congress. The recommendations call out the need for better information on ARs to support reservoir operation, among other things. Implementation could begin soon, aided by a CW3E supercomputer dedicated to ARs that can run state-of-the-art models and test artificial-intelligence analyses of data, in partnership with NWS.
In Portugal, where ARs also cause substantial flooding, scientists recently recommended that forecasters use the new ranking scale. Forecasters along the western coast of southern South America are considering adopting it as well. And someday soon you may see an AR icon as a standard part of your favorite weather app or forecast.
Better forecasts can increase society’s resilience in the face of climate change. In just the past five years major areas in the U.S. West have faced record drought followed by record floods, swings that climate scientists say will likely occur even more often in the future. These extremes seem to be fueled by stronger ARs that are then separated by longer, hotter dry periods.
AR forecasts are already helping water managers take advantage of impending precipitation and runoff. Consider the Lake Mendocino area, 100 miles north of San Francisco near the coast. Engineers created the lake in 1959 by damming the eastern branch of the Russian River to help control devastating downstream floods. The project also created a reservoir for the Sonoma County wine region and a hydropower plant. If storms push the lake beyond 68,000 acre-feet of water during January to March, managers typically release the excess through the dam. But if that level is exceeded only modestly during late March or April, when flood season ends, they keep the water for the dry summer. With climate change, however, in most years since about 2000 there has not been enough spring rain to refill the lake.
Given that trend, and a recent scientific and engineering project called Forecast-Informed Reservoir Operations, in 2021 the army corps adopted a five-year demonstration of this new approach at this reservoir. It allows operators, after a storm, to keep up to 11,000 acre-feet of extra water (the “buffer pool”) above the 68,000 acre-feet “conservation pool.” This option is reconsidered each day, as long as no AR is predicted for the next few days. If no strong AR occurs by the end of spring, this practice can retain enough water to supply 20,000 or more households for a year.
Early in January 2020 the new AR tools indicated a storm might hit. When it arrived, army corps reservoir operators kept the dam closed, allowing the lake to fill partway to the buffer pool limit. Another modest AR soon followed, and the operators retained the full 11,000 acre-feet. For the rest of the winter, if an AR was forecast, they would release extra lake water, but no other major storms arrived. The 11,000 acre-feet of water became extremely valuable because that summer the Russian River region north and south of the lake, all the way to San Francisco, was extremely dry. This approach enables water managers to more reliably provide water supply during drought conditions.
AR forecasts were also crucial in the Vancouver region during November 2021, following an autumn of heavy rain and mountain snow. Two back-to-back AR4s struck Vancouver, the mountains above it and greater British Columbia, dropping up to 11 inches of rain in two days. Heavy flooding and landslides shut down the major roads and rail lines to the city, cutting it off from the rest of Canada. The Port of Vancouver, the country’s largest, had to stop operations right in the middle of an unprecedented supply-chain crisis brought about by the COVID pandemic. AR forecasts helped officials decide to evacuate thousands of people.
Forecasts are also now available in places where ARs have not been widely recognized. In January 2022 a storm over the Gulf of Mexico reached “extreme” intensity, crossing the southeastern U.S. and fueling a dangerous nor’easter across the Northeast. In Antarctica, evidence suggests that ARs are bringing most of the new snow that falls on higher parts of the ice sheet, yet in March 2022 a warm, wet airstream was blamed for temperatures that were 72 degrees Fahrenheit above normal, bringing lots of snow inland but rain and snowmelt along the coast. ARs have been implicated in big recent melts and rains on the Greenland ice sheet, too.
Improving AR forecasts further will help scientists determine what role atmospheric rivers may play in future climate change. Until then, the focus for forecasters is to enhance predictions for local emergency and water planners, including the army corps and California’s Department of Water Resources, which have been innovative in developing and including AR information in decision-making. Perhaps an AR warning for an upcoming storm can be issued one day sooner, or the rain total can be predicted with even greater accuracy, getting people out of harm’s way or increasing water-supply reliability for inevitable dry days to come.